Introduction
High-volume forging is a manufacturing process that involves applying compressive forces to heated metal billets or slugs to deform them into desired shapes and sizes. This process is widely used in various industries, including automotive, aerospace, and construction, to produce large quantities of high-strength, durable components with complex geometries. The importance of high-volume forging lies in its ability to create parts with superior mechanical properties, such as increased strength, toughness, and fatigue resistance, compared to other manufacturing methods like casting or machining.
Key Stages in the High-Volume Forging Process
1. Pre-Forge Preparation
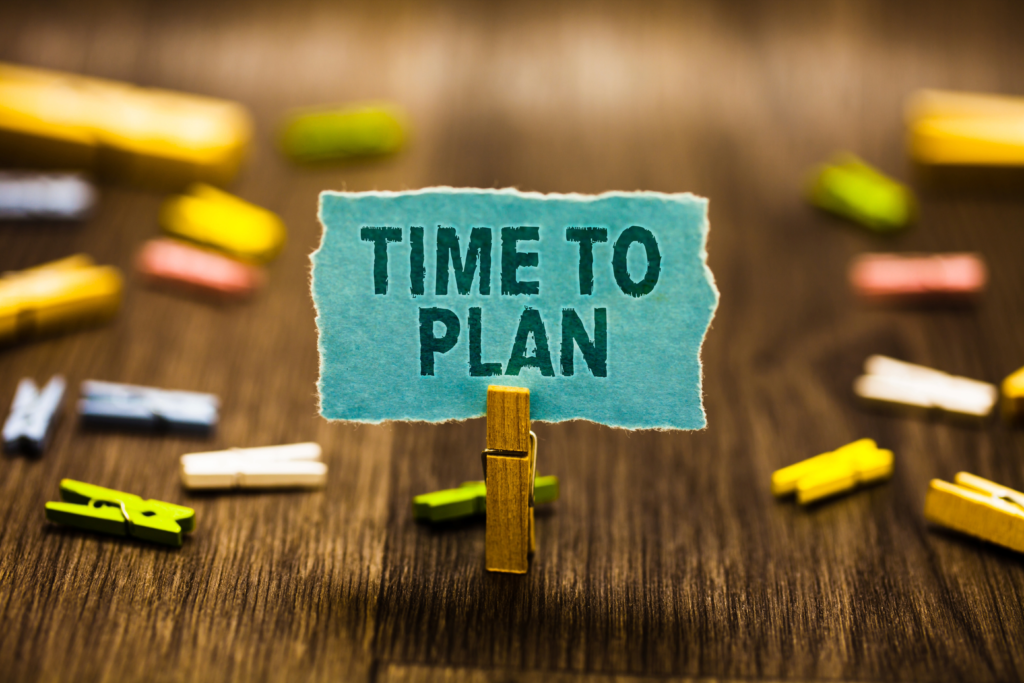
The pre-forge preparation stage involves selecting the appropriate raw materials, typically in the form of billets or slugs made from various metals like steel, aluminum, or titanium alloys. The billets are cut and sized according to the desired final product dimensions. Preheating the billets to a specific temperature range is crucial to ensure proper deformation during the forging process.
• Selection of Raw Materials: The initial step involves choosing high-quality raw materials, such as billets or slugs. The material selection depends on the desired properties of the final product. Common materials used in forging include steel, aluminum, titanium, and various alloys.
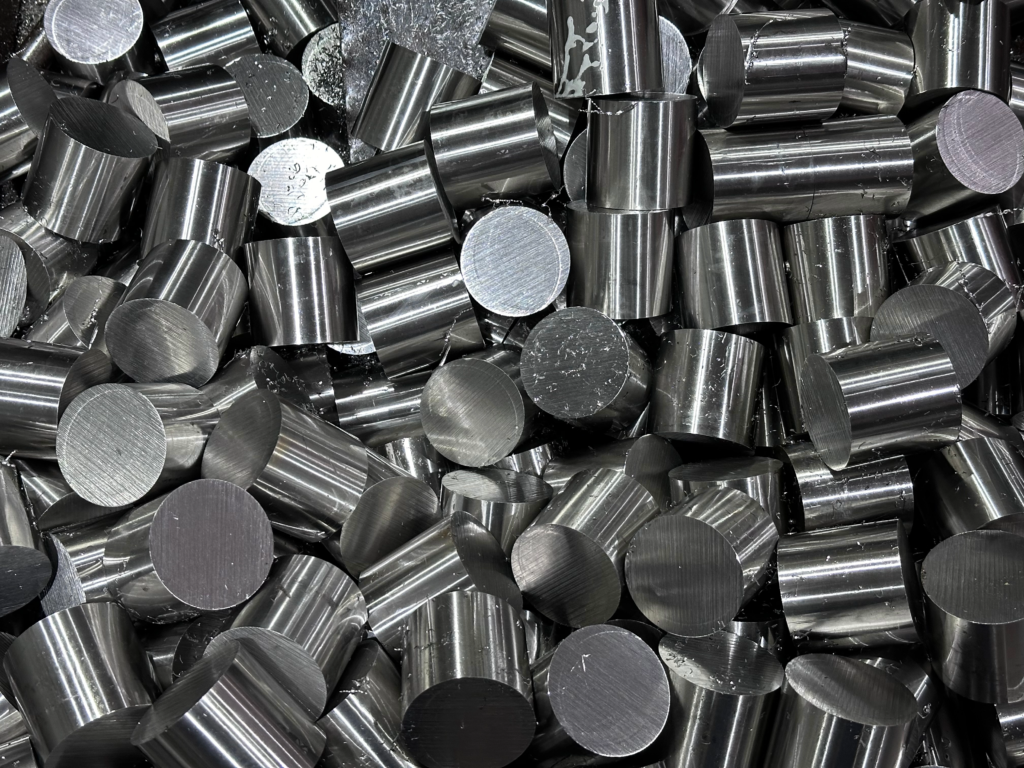
• Billet Cutting and Sizing: Once the raw material is selected, it is cut into billets or slugs of specific sizes. This step ensures that each piece of material is of the correct dimensions for forging.
• Preheating Requirements: Preheating the billets is essential to achieve the necessary plasticity for forging. The preheating temperature depends on the material being forged and the desired properties of the final product.
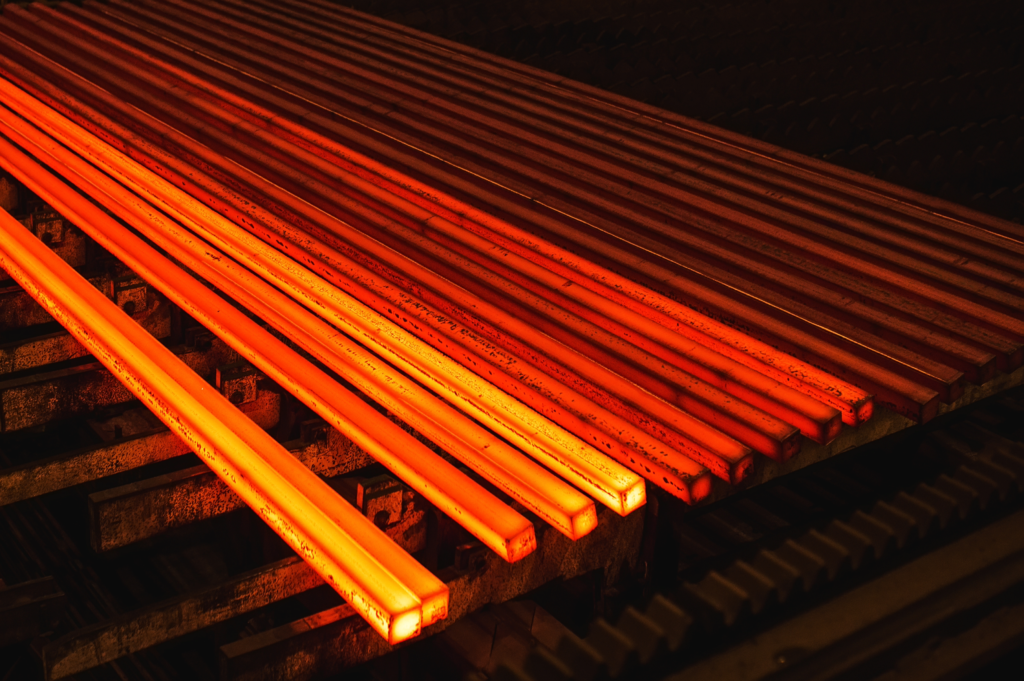
2. Heating Methods
Heating the billets to the required temperature is a critical step in the forging process. Two common heating methods are:
- Induction heating: This method uses an alternating electromagnetic field to induce eddy currents within the billet, causing it to heat up rapidly and uniformly. Induction heating is energy-efficient and provides precise temperature control[1].
- Furnace heating: In this method, billets are heated in a furnace, typically fueled by gas or electricity. Furnace heating is suitable for large-scale production but may result in temperature variations within the billet[2].
Other heating techniques, such as resistance heating or direct flame heating, may also be employed depending on the specific requirements and production volumes.
3. Forging Operations
The forging operation involves applying compressive forces to the heated billets to deform them into the desired shapes. Two main types of forging operations are:
- Open-die forging: In this process, the billet is deformed between flat or simple-shaped dies, allowing for a wide range of shapes and sizes to be produced. Open-die forging is often used for large or irregularly shaped components[3].
- Closed-die forging: This method involves using a set of pre-machined dies with cavities that match the desired final shape of the component. Closed-die forging is suitable for producing complex geometries with tight tolerances and is commonly used for high-volume production.
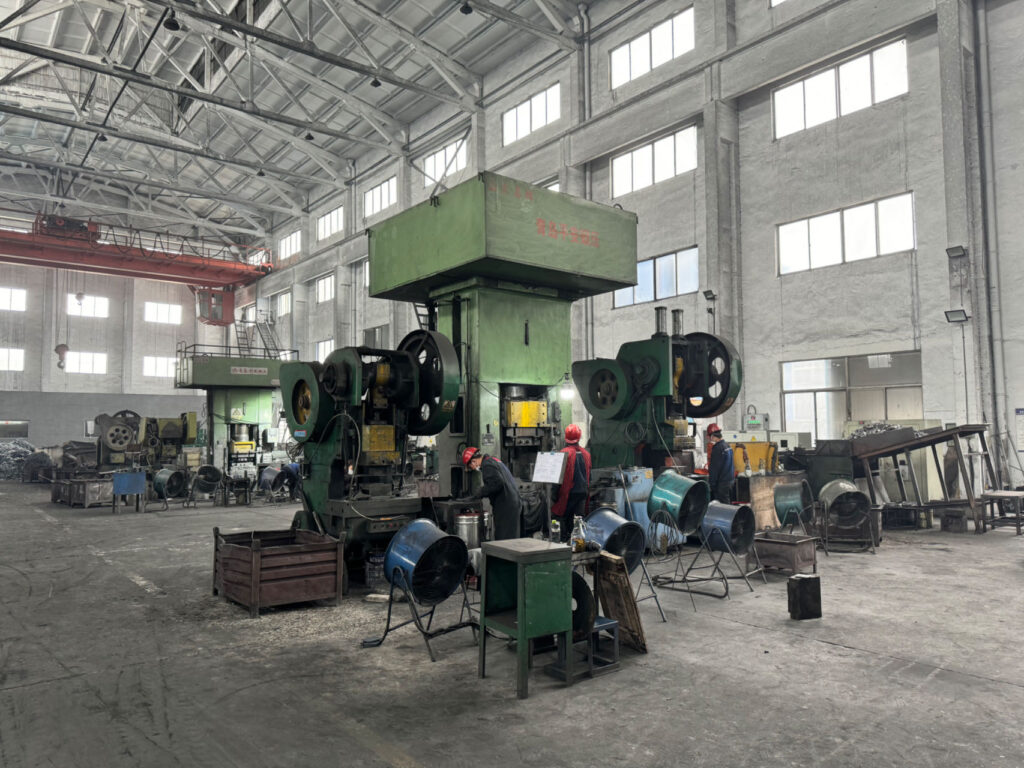
The selection of forging presses or hammers depends on factors such as the required force, production volume, and component size. Deformation mechanics, including factors like strain rate, temperature, and material flow, play a crucial role in achieving the desired final shape and properties.
4. Die Design and Manufacturing
Die design is a critical aspect of the forging process, as it directly impacts the quality and accuracy of the final product. The dies are typically made from high-strength, wear-resistant materials like tool steels or ceramics to withstand the extreme pressures and temperatures involved in forging.
The die design process involves several steps, including:
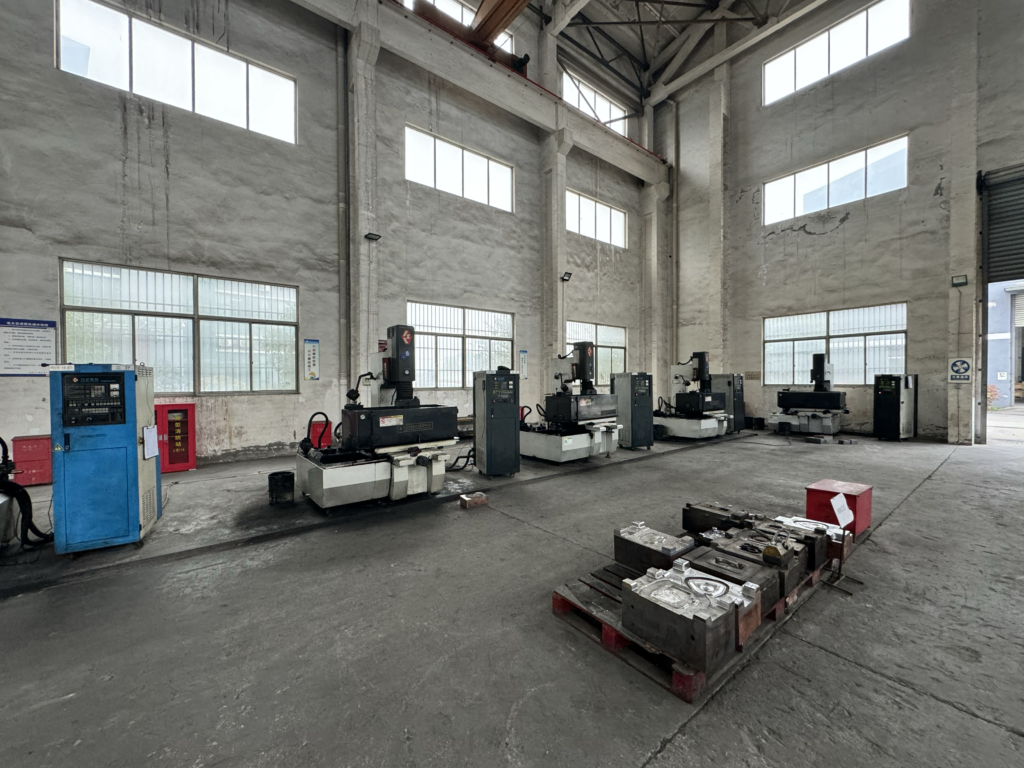
- Computer-aided design (CAD) modeling of the desired component geometry
- Finite element analysis (FEA) to simulate the forging process and optimize die design
- Die manufacturing using advanced techniques like computer numerical control (CNC) machining or electrical discharge machining (EDM)
- Heat treatment and surface finishing of the dies to enhance their durability and performance
5. Lubrication and Temperature Control
Lubrication plays a crucial role in the forging process by reducing friction between the billet and the dies, preventing material adhesion, and facilitating material flow. Various lubricants, such as graphite-based compounds or synthetic oils, are applied to the dies and billets using techniques like spraying or dipping.
Maintaining optimal forging temperatures is essential for achieving the desired material properties and preventing defects. Temperature control is achieved through precise heating and cooling cycles, as well as monitoring and adjusting the forging parameters throughout the process.
6. Forging Process Execution
During the forging process execution, the heated billets are subjected to compressive forces applied by the forging press or hammer. The deformation of the billet into the desired shape is achieved through a series of controlled forging operations, which may involve multiple strikes or passes through the dies.
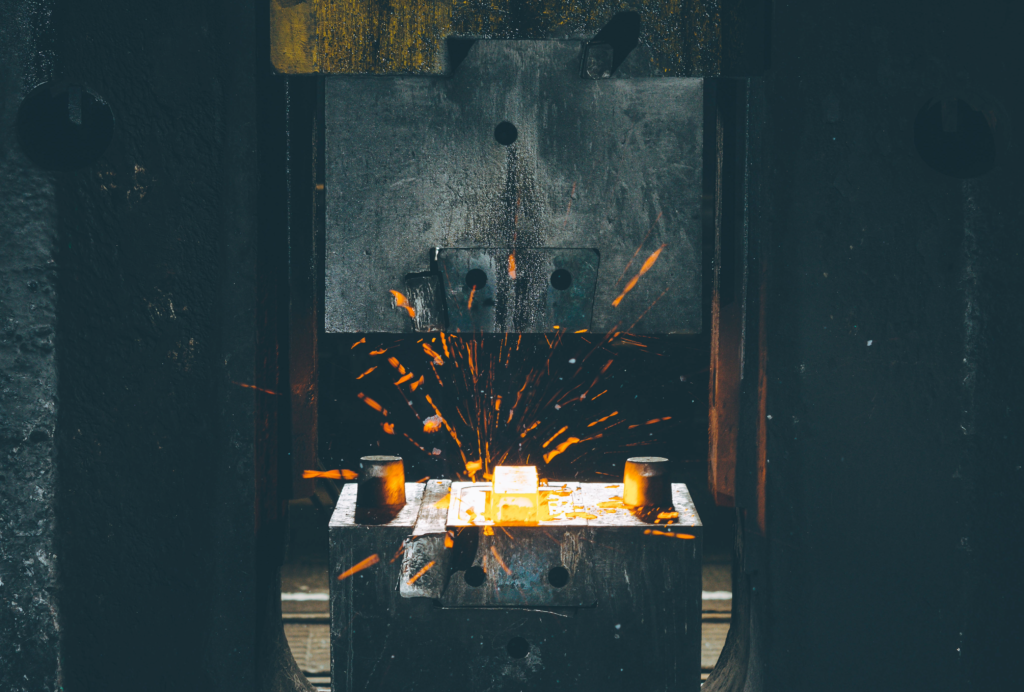
Achieving tight tolerances and complex geometries often requires specialized forging techniques, such as precision forging, isothermal forging, or hot die forging. These techniques involve precise control of temperature, deformation rates, and die design to ensure consistent and accurate results.
7. Cooling and Heat Treatment
After the forging process, the components undergo controlled cooling to prevent defects and achieve the desired microstructure and mechanical properties. Various cooling methods, such as air cooling, water quenching, or controlled atmosphere cooling, may be employed depending on the material and application requirements.
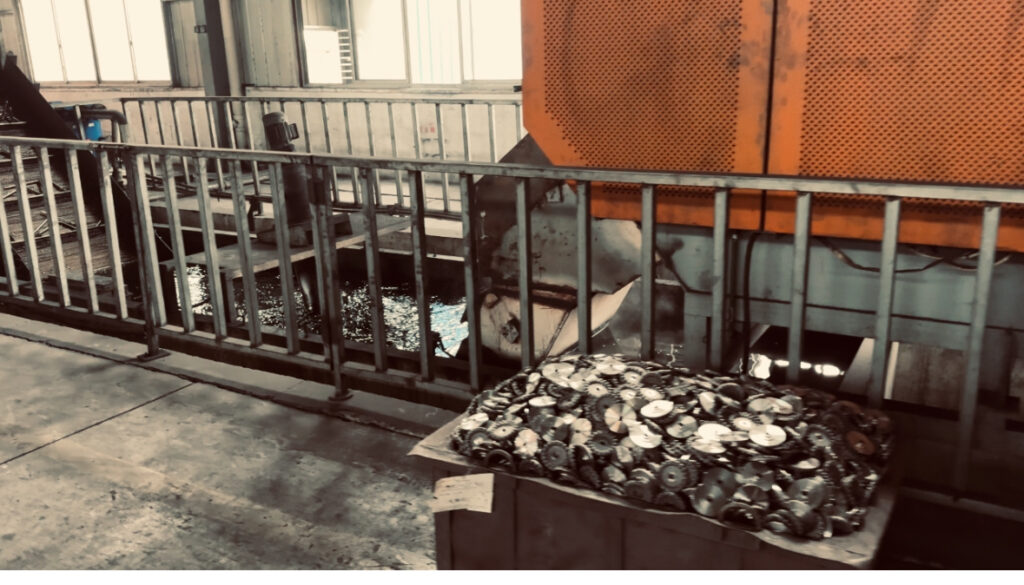
Heat treatment processes, such as annealing, normalizing, or quenching and tempering, are often performed to enhance the mechanical properties of the forged components. These processes involve carefully controlled heating and cooling cycles to modify the microstructure and achieve the desired combination of strength, ductility, and toughness.
Stress relief and hardening techniques, like shot peening or nitriding, may also be applied to improve the surface properties and fatigue life of the forged components.
8. Trimming and Finishing
After the forging and heat treatment processes, excess material, known as flash or fins, is removed from the components through trimming operations. This can be achieved using various methods, such as machining, grinding, or shearing, depending on the component geometry and material.
Surface finishing processes, like shot blasting, vibratory finishing, or polishing, may be applied to improve the surface quality and appearance of the forged components. These processes can also enhance fatigue life and corrosion resistance by removing surface defects and improving surface roughness.
Final inspection and quality control procedures are performed to ensure that the forged components meet the required specifications and tolerances before being released for use or further processing.
Post-Forging Processes
After the forging process, the components may undergo additional post-forging processes, such as:
- Quality inspection and testing: Various non-destructive testing (NDT) methods, like ultrasonic testing, magnetic particle inspection, or dye penetrant testing, are employed to detect internal defects or flaws in the forged components.
- Dimensional and metallurgical analysis: Precise dimensional measurements and metallurgical analysis, including microstructure examination and mechanical property testing, are conducted to ensure compliance with specifications and quality standards.
Quality Control Measures
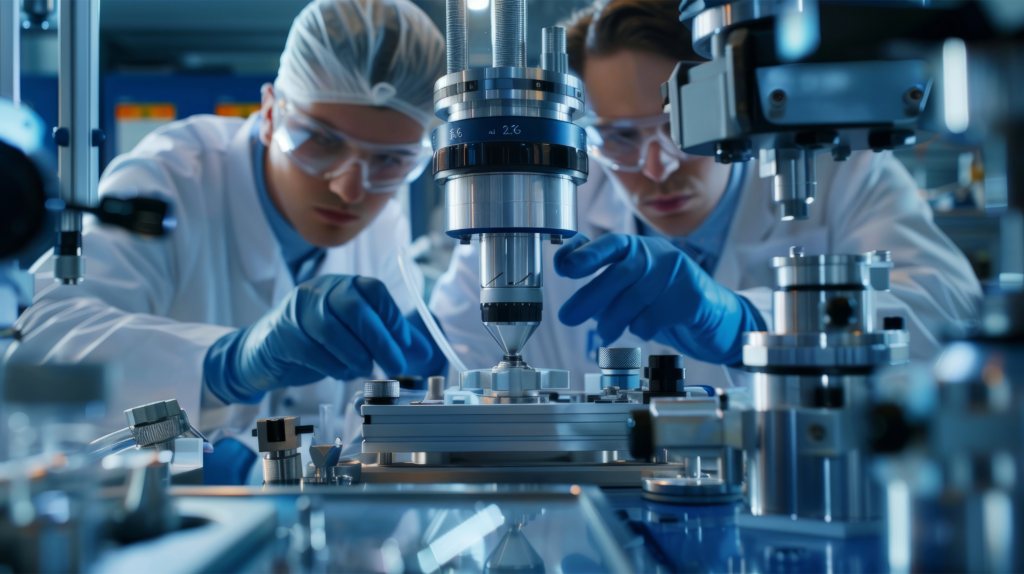
Quality control is of utmost importance in the high-volume forging process to ensure consistent product quality and reliability. Common defects in forged components include surface defects, internal cracks, porosity, and dimensional inaccuracies.
To prevent and mitigate these defects, various quality control measures are implemented, such as:
- Strict adherence to established forging parameters and procedures
- Regular maintenance and calibration of forging equipment
- Comprehensive inspection protocols and standards, such as ASTM or ISO standards
- Statistical process control (SPC) techniques to monitor and control process variability
- Continuous improvement initiatives, such as root cause analysis and corrective action plans
Innovations and Technology in High-Volume Forging
The high-volume forging industry is continuously evolving, driven by advancements in technology and the need for improved efficiency, quality, and cost-effectiveness. Some notable innovations and technologies in this field include:
- Automation and robotics: The integration of automated systems and robotic handling has increased productivity, reduced labor costs, and improved consistency in the forging process.
- Simulation and modeling software: Advanced computer simulations and finite element modeling tools are used to optimize die design, predict material flow, and analyze stress and strain distributions, leading to improved product quality and reduced development time.
- Advanced heating technologies: Induction heating systems with improved power control and efficiency, as well as advanced furnace designs with better temperature uniformity and energy efficiency, have been developed to enhance the heating process.
- Smart manufacturing and Industry 4.0: The adoption of smart manufacturing principles, including the integration of sensors, data analytics, and machine learning, has enabled real-time process monitoring, predictive maintenance, and optimized production planning.
Case Studies and Applications
High-volume forging finds applications in various industries due to its ability to produce high-strength, durable components with complex geometries. Some real-world examples and benefits observed include:
- Automotive industry: Forged components like crankshafts, connecting rods, and suspension parts are widely used in automotive applications due to their superior strength-to-weight ratio and fatigue resistance, contributing to improved vehicle performance and fuel efficiency.
- Aerospace industry: Forged components, such as landing gear components, turbine discs, and structural parts, are essential in aerospace applications due to their high strength, toughness, and resistance to extreme operating conditions.
- Construction and heavy machinery: Forged components like gears, shafts, and structural components are used in construction equipment, mining machinery, and other heavy-duty applications due to their durability and ability to withstand high loads and harsh environments.
Conclusion
The high-volume forging process is a critical manufacturing technique that enables the production of high-strength, durable components with complex geometries in various industries. From pre-forge preparation to post-forging processes, each stage plays a crucial role in ensuring the quality and reliability of the final product.
As technology continues to advance, innovations in areas such as automation, simulation, and smart manufacturing are expected to further enhance the efficiency, precision, and cost-effectiveness of the high-volume forging process. Additionally, ongoing research and development efforts will likely lead to the development of new materials, processes, and applications, further expanding the capabilities and applications of high-volume forging in the future.
Citations: [1] https://starpathrail.com/rail-clip/ [2] https://starpathrail.com/tie-plate/ [3] https://starpathrail.com/arema-improved-anchor/